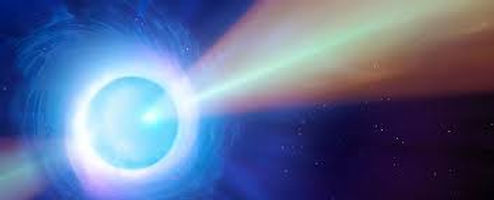

Margaret Guo '23
How Can We Use Pulsars to Detect Gravitational Waves?
Best article
​What is a pulsar?
Pulsars are a type of highly magnetized, rapidly rotating neutron star. But what is a neutron star? A neutron star is the remnant of a much larger star that collapses inwards (supernova) with such force that the individual atoms in the star collapse, such that the electrons are combined with protons. The result is a star made entirely of neutrons, packed VERY densely together. A certain type of neutron star, called a pulsar (shown below), emits radio waves (and sometimes Gamma or X-ray radiation too) from two magnetic poles. As they are formed from the supernova explosions of massive stars, pulsars retain their angular momentum while shrinking in size, causing an increase in spin frequency. This can be compared to a figure skater pulling in their arms in to spin faster. Angular momentum must be conserved, so as the radius of the star decreases, its velocity must increase. Pulsars are highly magnetized, very dense, and spin rapidly. Since the magnetic and rotational axes of a pulsar are not necessarily aligned, its beam of radiation comes in and out of our line of sight, causing us to detect a “pulse”. With each rotation, we detect a pulse of radiation. These pulses are very regular, like a clock.
​
Diagram of a pulsar
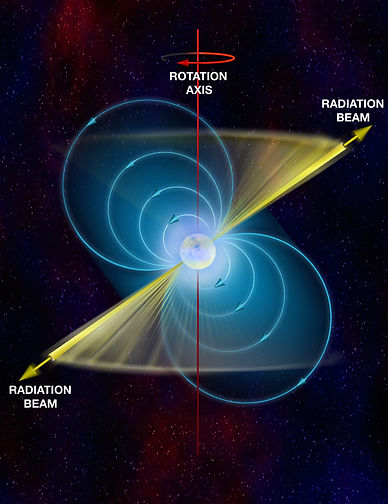
So then, what is a gravitational wave?
Einstein’s general relativity predicts that the movement of massive bodies should create some sort of ripple in the fabric of spacetime. Astronomers have been able to detect these waves numerous times through observations of pulsars, providing strong evidence in support of Einstein’s General Relativity. While the gravity we feel on Earth can be thought of as a dent in spacetime, a gravitational wave is more like a moving ripple. Gravitational waves have extremely long wavelengths. Only the most violent and extreme phenomena can create significant gravitational waves. Think supernova explosions, collisions of neutron stars, and black holes merging. As a gravitational wave passes, it will cause objects to shrink and stretch. The weight of objects, the distance between them, and their densities will all be affected by passing gravitational waves. However, gravitational waves from faraway events have very little effect by the time they reach us on Earth, which makes them tricky to detect directly. Based on the Big Bang model, there should be a gravitational wave background from the random and extreme events of the early universe. The waves would have to be created about 10^-35 seconds after the Big Bang, way before the cosmic microwave background. Due to the expansion of the universe, these waves would be drastically stretched out.
​
How are pulsars and gravitational waves connected?
The pulses we detect from a pulsar are very regular. But, the passing of a gravitational wave (which causes increase/decrease of distances) can cause us to detect a pulse early or late. This type of disruption in the period of a pulsar is the key to detecting gravitational waves using pulsars. We use time of arrival (ToA) of these pulses to do pulsar timing, which is the method used to find disruptions in the ToAs of pulses. Basically, once we are able to calculate when a pulse SHOULD arrive, we will be able to track any differences between the expected and observed times of arrival. The difference between the observed and computed arrival time of pulses is known as a timing residual (residual = observed ToA – computed ToA). These timing residuals may be very small; after years of observing, there may only be a difference of a few tens of nanoseconds.
​
To determine if the disturbances detected are actually caused by gravitational waves, we must determine if our findings correspond with properties that only gravitational waves show. This requires a pulsar timing array, which is a set of pulsars that can be observed to find correlations in their timing residuals. Gravitational waves cause changes in both the pulsars we observe and our telescopes on earth. The effect of a gravitational wave on an earth telescope, the “Earth-term”, follows a specific pattern of correlation between pulsars based on angular separation of two pulsars measured. This correlation is unique to gravitational waves and helps us distinguish the effect of gravitational waves from other radio noise in the universe. If the timing residuals are caused by gravitational waves, the correlation of timing residuals is plotted against angular separation between pulsars should form the Hellings-Downs curve, shown below.
​
If we find that the residuals of a pulsar timing array fall along the Hellings-Downs curve, we know that the disturbance must be gravitational waves from a stochastic gravitational wave background as predicted by general relativity.
​
Since the first detection of gravitational waves using pulsars in 1974, there have been major advancements in gravitational wave and pulsar research. Many collaborations including NANOGrav, the North American Nanohertz Observatory for Gravitational Waves, look to further understand gravitational waves through study of pulsars. More recently in 2015, we were able to detect gravitational waves directly for the first time with the Laser Interferometer Gravitational-Wave Observatory (LIGO).